Research
The Choo lab focuses on micro-/nanoscale optics, micro-/nanofabrication, advanced microscopy/nanoscopy, and their applications to biological/biomedical imaging. We explore the extremes of micro-/nanoscale sciences, push the boundaries of state-of-the-art micro-/nanotechnologies, and engineer solutions to some of the major technical challenges that our society faces.
Some of our funding sources include the Heritage Medical Research Institute (HMRI), the National Institutes of Health (NIH), AMGEN, SAMSUNG, and the Powell Foundation.
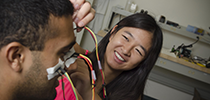
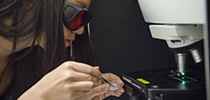
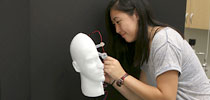
Research Areas
- Nanophotonics-based, micro-/nanoscale biomedical implants with optical readouts to improve the management of glaucoma
- Nanoscale single-molecule diagnosis platforms for in vivo and ex vivo monitoring of biological analytes such as glucose and other bio-molecules
- Bio-inspired sensor and imager designs
- Label-free imaging techniques for studying the pathogenesis of major human diseases
Implantable Intraocular Pressure Sensor with Remote Optical Readout: The SEM/photo images and the conceptual illustration (bottom) of the world’s smallest implantable intraocular pressure sensor with the largest readout distance: the sensor measures 600-800 μm in diameter (about 6-8 hair strands) while the pressure can be detected at > 10 cm. The system uses a broadband light source such as a tungsten or halogen bulb. The work has been nominated for the best paper award at the IEEE MEMS 2016 Conference in Shanghai, China.
Microelectromechanical Systems for Biomedical Applications: The SEM/photo images show micromirrors and microelenses integrated with electrostatioc acutators. The micromirrors can function as microscanners that can either read in the image or write patterns. In this example, the micromirrors replicated the microscale patterns on a US dime by emulating an ablation system. And, the individually addressed actuated microlenses shown next to the mirrors can vastly improved the measurement range of Shack-Hartmann sensors widely used to characterize the optical aberrations of human eyes.
Energy Harvesters for Deep Brain Stimulators: Neuro-stimulation for epilepsy, chronic pain, and depression by using electronic implants is one of the fast growing sectors in medical industry, but it requires battery replacement. We are exploring the use of vocal vibrations as a potential energy source. Vocal vibrations provide tunable, well-defined vibration frequencies and amplitudes and propagate throughout head and neck. Our initial study shows that the approach can generate a sufficient level of power for medical electronic implants. A part of this work has been featured in the science section of the Economist.
- Ultra-fast, energy-efficient, nanoscale optoelectronic components for future telecommunication and computing applications
- Present effort concentrated on engineering on-chip nanofocusing devices with strong enhancement, high throughput/efficiency, and tunability
3D Nanoplasmonic Photon Compressor for Highly Efficient Nanofocusing: The development of techniques for confining photons efficiently on the deep sub-wavelength spatial scale will revolutionize scientific research and engineering practices. The efficient coupling of light into extremely small nanofocusing devices has posed a major challenge in on-chip nanophotonics due to the need to overcome various loss mechanisms and the on-chip nanofabrication challenges. Here we demonstrate, experimentally, the achievement of highly efficient nanofocusing in an Au-SiO2-Au gap plasmon waveguide using a carefully engineered 3D taper. The dimensions of the SiO2 layer, perpendicular to the direction of wave propagation, tapered linearly below 100 nm. Our simulations suggested that the 3D linear-tapering approach could focus 830 nm light into a 2-by-5 nm2 area with ≤3 dB loss and an intensity enhancement of 3.0 × 104. In a two-photon luminescence measurement, our device achieved an intensity enhancement of 400 within a 14-by-80 nm2 area and transmittance of 74 %.
3D Nanoplasmonic Photon Cavity with Plasmonic Crystals for Ultimate Nanoscale Light Confinement: We have investigated the properties of a deep sub-wavelength-scale on-chip optical cavity composed of a highly efficient metal-insulator-metal 3D-tapered plasmonic nanofocusing waveguide and easily tailorable metal-insulator-metal plasmonic crystals. Simulation studies indicated that this configuration with a thin dielectric layer as 10x15 nm2 in area could efficiently confine electromagnetic waves on the nanometer length scale through a field intensity enhancement of 7 × 103 and a Purcell enhancement of 8 × 103 within a volume of 1.4 × 10–5 λ03. The overall focusing efficiency was calculated to be around 85 %.
3D Microfiber Cavity with Lattice-Varying Holes for Ultimate Balance in Mode Volume and Qualtiy Factor: We investigated a novel approach to confine light in a silica microfiber (MF) cavity using a lattice constant-varying nanohole array. In our simulation study, the MF cavity is integrated with an optimally designed nanohole array, and produces Q-factors as high as 7×105 in the near-infrared region. The mode volume of the cavity remains close to λe3, a much smaller value than those previously reported for MF-based high-Q resonant structures.
Radiation-Engineered Metal-Clad Nanocavity for Highly Efficient Directional Coupling: We have investigated a cladding engineering method that flexibly modifies the radiation patterns and rates of metal-clad nanoscale optical cavity. Optimally adjusting the cladding symmetry of the metal-clad nanoscale optical cavity modifies the modal symmetry and produces highly directional radiation that leads to 90% coupling efficiency into an integrated waveguide. In addition, the radiation rate of the cavity mode can be matched to its absorption rate by adjusting the thickness of the bottom-cladding layer. This approach optimizes the energy-flow rate from the waveguide and maximizes the energy confined inside the nanoscale optical cavity.
Sloped-Sidewall Half-Sized Metal-Clad Nanocavity (Nanohorn) with Much Improved Mode Confinement: We have investigated in simulation a novel nano-horn metal-clad optical cavity that is approximately 0.8 micron in height. The cavity achieves a Q factor of ~1000 (which is about six times higher than the Q factors of previously reported approaches) and has an effective volume of 0.31(λ/n)3.
- Bio-inspired/biomimetic optical structures
- Bio-inspired/biomimetic decision-making processes
For more information, please visit our publication page. We will update our publication list as soon as the relevant work is published. Or simply contact us. We have more projects in progress and manuscripts under preparation that are not listed on our webpage.